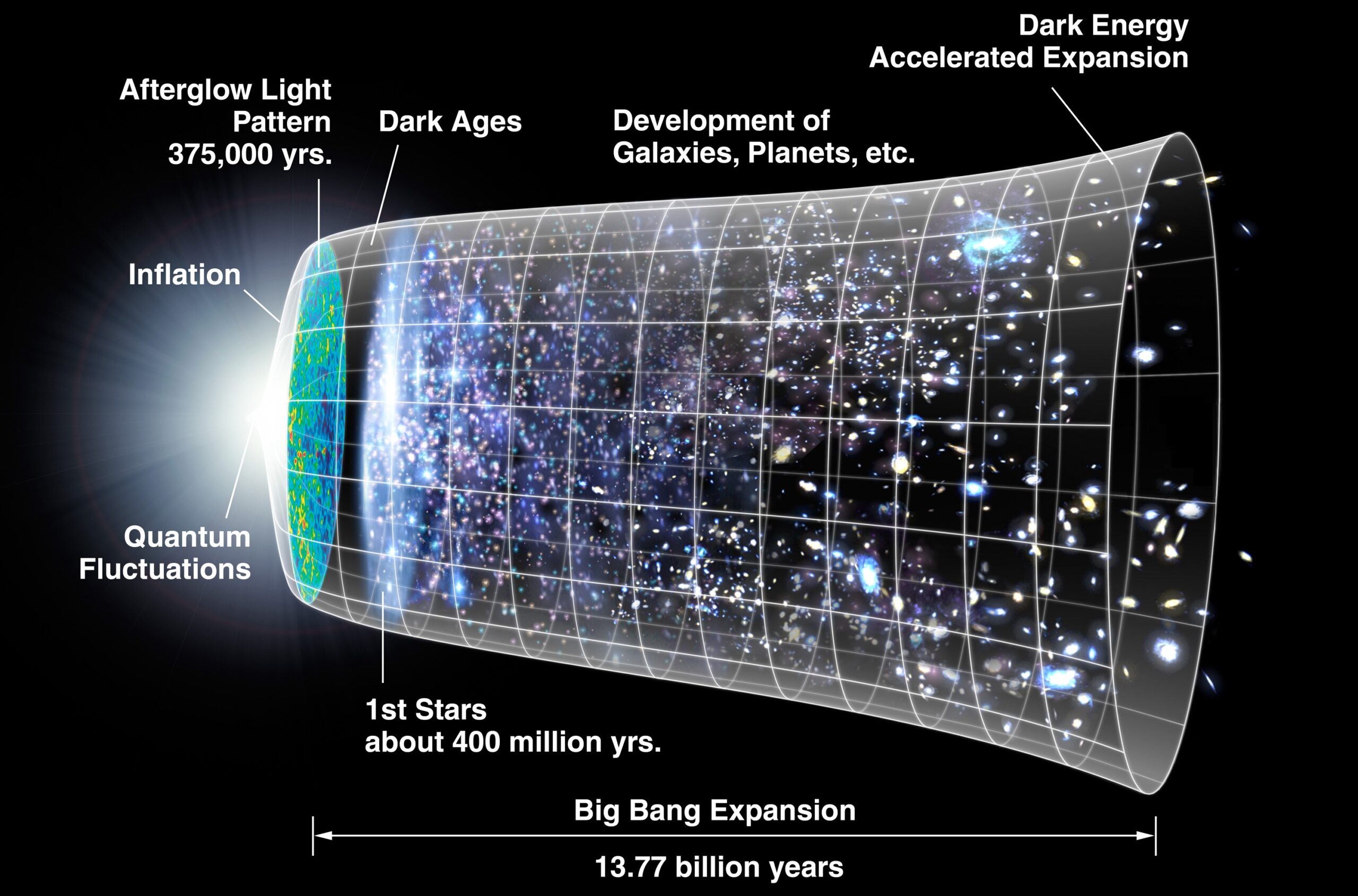
TImeline of the universe’s evolution from its primordial beginnings until today. Note the CMB as the “Afterglow Light Pattern” at 375,000 years followed by the formation of the first stars at about 400 million years. These points on the timeline are where we discuss two foundational points, supporting our assertion that the universe’s age is still 13.77 Gyr.
Using data and imagery obtained with the JWST, a recent study claims the universe is now 26.7 billion years old. We will unequivocally show that the age of the universe is still 13.77 Gyr.
One of the principal reasons the JWST mission was conceived in the first place was to test existing theories and current models of cosmology, dark matter and the large-scale expansion of the universe. We knew JWST would answer some existing questions while leading to new ones. Although the Hubble Space Telescope has contributed mightily to our body of knowledge, it has reached its limitations in just how far back into the cosmic sands of time it can probe. Why?
The Limitations of HST
Although the Hubble Space Telescope has a Near Infrared camera (NICMOS), this camera has been out of operation since 2008 with its functionality superseded by the Wide Field Camera 3. Yes, HST can “see” in the infrared, but its limitations here are also affected by its size. A telescope’s resolving power (the ability to see and distinguish fine detail) and how deep (how faint) it can “see” is governed by its physical diameter and the wavelength of light being observed (this last limitation applies only to the resolving power). Although HST can observe objects in the infrared, it is limited by its 2.4 meter size compared to JWST’s 6.5 meter size.
It is important to note here that HST’s optical performance is unparalleled, approached only by ground-based telescopes equipped with adaptive optics. JWST is exclusively an Infrared telescope, with the only optical overlap occurring at 600 nanometers (red light) with the NIRCam instrument.
So why do we need JWST, and why can’t Hubble’s infrared Capability answer these fundamental questions?
In 1929 Edwin Hubble published his watershed study, for the first time providing a relationship between distance and recessional velocity. Known as the Hubble Constant, it not only verified what had been predicted 2 years earlier in 1927 by Georges Lemaître, that the universe was expanding and this expansion rate increased with distance. The conclusion: the more distant the object, the greater its recessional velocity.
This conclusion had profound implications, putting upper constraints on when the universe began and how large it is.
Since Hubble’s initial determination in 1929, his constant has been refined to where it is today. With a value of about 70 km/sec/megaparsec, an object is receding 70 km/sec faster for every million parsecs distance (a parsec is ~ 3.26 light years). Since this is a “rate of expansion”, we can extrapolate backwards in time to determine its age. Using the accepted value of Hubble’s Constant, this extrapolation yields an age of 13.77 billion years. This value has been refined using many independent methods and lines of inquiry over the intervening 94 years to the value it is today, all with a high degree of concordance.
Light as the Universal Speed Limit
Because of the finite speed of light, the universal speed limit, no matter where or how far away we look, we’re always looking back in time. The more distant the object, the further back in time we’re looking – we’re seeing an object as it was, not as it is. And this is where we answer the question: why do we need JWST.
The faster an object is moving relative to an observer, the more its light will be shifted towards the blue (shorter wavelengths) or to the red (longer wavelengths). Light from objects approaching an observer will be shifted towards the blue. Light from objects receding from an observer will be shifted towards the red. This light is said to be red-shifted or blue-shifted.
Observations with HST can bring us back only so far. In order to “see” how the universe was at the dawn of cosmic history, we have to “see further” than what was theoretically possible with HST. It’s not that HST isn’t capable of observing these objects. The problem is their distance is so great, their visible light is “red shifted” into the infrared part of the electromagnetic spectrum and thus beyond detection by HST. Thus arose the need for an instrument capable of detecting these beacons from the dawn of cosmic time.
The Early Universe
The primordial universe was a very different place than it is today. Known as the Primordial Abundance, it began with 73% Hydrogen and 25% Helium, with 2% trace amounts of Lithium and Beryllium. Slowly and inexorably over time, stellar nucleosynthesis, the nuclear fusion process that powers all stars, changed that abundance to where it is today to include all the elements up through Uranium. It has been postulated that Population III stars, the very first stars that formed 380 million years after the initial inflationary event known as “The Big Bang”, included ultra-high mass, super luminous stars.
These stars went through their compliment of hydrogen fuel at prodigious rates, ultimately ending their very short lives spectacularly as Type-II supernovae, the process by which the heavy-elements built up internally enrich the interstellar medium. Using the just released data from NASA’s ERO program for JWST, we published our own study last year that strongly suggests this was the case in the early universe.
Multiple Lines of Inquiry
There are multiple lines of inquiry with a high-degree of concordance that confirm the age of the universe at 13.77 billion years. To claim that this value is now 26.7 billion years based on a single outcome from a single study is to upend almost a century of study that included multiple Nobel Prizes, multiple NASA missions along with painstaking research by countless, thousands of individuals.
Type Ia Supernovae
We assert that no Type Ia supernovae will be found in galaxies with a redshift > 4. Our previous study using JWST ERO program data discusses this in detail.
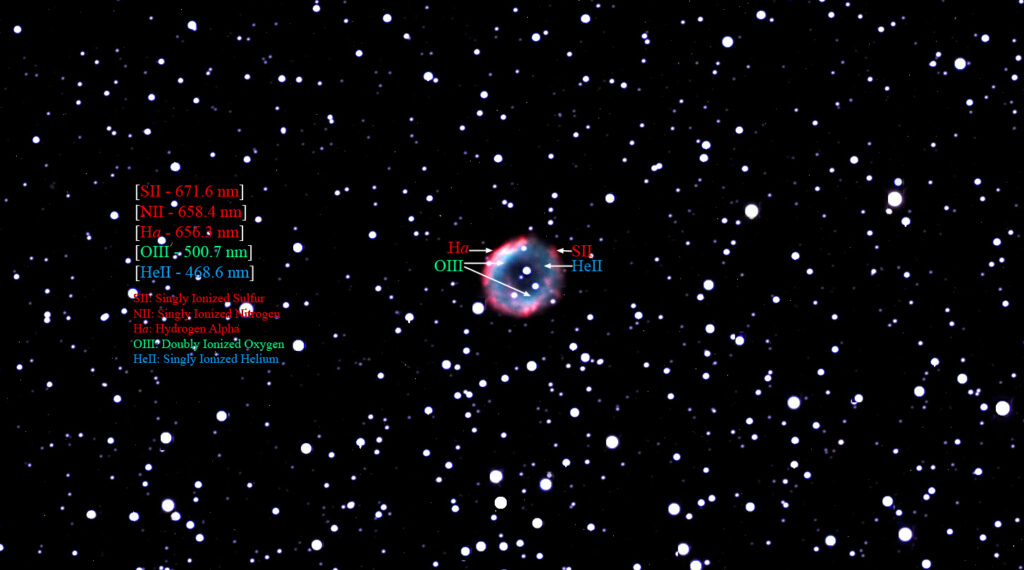
Planetary Nebula NGC-2438 illustrating the presence of Oxygen, Hydrogen and Helium. Image credit: the author.
Nucleosynthesis, the building of heavier elements in the stellar core as the byproduct of nuclear fusion reactions using lighter elements, ends with the Triple-Alpha (Helium burning) process in stars below 4 solar masses. This process uses helium nuclei in a 2-stage or, in some instances, a 3-stage process in the production of carbon and oxygen in the stellar core. The end-state here is a planetary nebula as an expanding shell of gas of what used to be the star’s outer layers.
The hot white dwarf remains at the center, ionizing this expanding shell of gas, causing it to shine. Over 10s of thousands of years, the expanding shell of gas dissipates, getting ever more tenuous, eventually fading away into the interstellar medium. The white dwarf remains, slowly radiating away any remaining heat over the course of billions of years. This is the end-state for our sun and stars below 4 solar masses.
If it lived out its life as a single star, this is how stars below 4 solar-masses end. If, however, the star was a member of a binary system or if it captured another star as a result of its proper motion through the galaxy, its end will be quite different.
Type Ia supernovae (pl) occur as the end-state for some white-dwarf stars in the final phase of their evolution – not all but some.
Carbon Burning Threshold
A star below 4 solar masses lacks the mass necessary to raise the core temperature above 600 million Kelvin, the ignition threshold for Carbon burning. Stars above this mass continue with the highest mass stars (> 8 solar masses) ending as Type II supernovae when they reach Iron and Nickel as the last stage in the Silicon burning cycle. Thus, white dwarfs are the remnants of stars below 4-solar masses.
The Standard Solar model teaches us that the stellar core contains approximately 70% of the star’s total mass. Thus, the white dwarf remnant that will one day be what remains of our sun will be, by definition, 0.70 solar masses. Over the course of the intervening 10 billion years of the sun’s productive lifetime, fusing hydrogen into helium, then helium into carbon and oxygen, will have compressed its core to about the size of the earth. The density and gravity of this compressed core will have dramatically increased, and any star in orbit around it will lose material, spiraling onto the surface of the white dwarf.
Critical Mass
When, in a binary system containing a parasitic white dwarf, the accumulated mass of the white dwarf reaches the Chandrasekhar limit of 1.39 solar masses, the “critical mass”, a runaway chain reaction ensues. Beginning with the accumulated stellar material from its companion (mostly hydrogen and helium), runaway nuclear fusion begins on the white dwarf’s surface, first consuming the accumulated surface material, then the carbon and oxygen. This process continues right up the nuclear ladder, through the Silicon burning cycle, ending with the production of Iron and Nickel. The resulting explosion completely obliterates the star in a Type Ia supernova, the singular most powerful event in the universe.
Standard Candles
These supernovae are critical standard candles, rungs on the cosmological distance ladder. Their intrinsic (fundamental) luminosity is reliable and consistent -because of the critical mass threshold necessary for the event. When we detect these supernovae, we know how fundamentally bright they are and thus can reliably determine their distance. This reliability led to the success of the 2011 study that shed new light on the expanding universe and resulted in a Nobel Prize for 3 recipients.
Detection
Unlike the spectra of Type II (core-collapse) supernovae, the telltale spectra of Type Ia supernovae contain no light elements (hydrogen or helium), as these elements were completely consumed in the ensuing maelstrom.
We’re discussing cosmology and the large-scale evolution of the universe here, so why is this important?
Stellar Evolution and a Redshift of 4
In order for a Type Ia supernova to occur, there must be a white dwarf progenitor star. These stars represent the final evolutionary stage for stars below 4 solar masses. They must live out their lives to the end – we have to wait for them to exhaust their compliment of hydrogen and run through the helium that was built up (the Triple Alpha process) before the white dwarf phase. For a solar-mass star, this takes about 12 billion years. A 4-solar mass star reaches this point in about 400 million years. Thus, the only white-dwarf progenitors would be those from stars whose initial mass was between 3 and 4 solar masses. For lower-mass stars, there simply wasn’t enough time for their white dwarfs to form in the very young universe.
For a solar-mass star to reach its white dwarf phase, we would need almost the current age of the universe as a wait time!
Using the stellar evolution of 3-4 solar-mass stars, we constrain the redshift when Type Ia supernovae would first appear. This constraint corresponds to a “look-back time” of 13 billion years (z = 4) as follows:
13.8 Billion years – (380 million years for the first stars to form + 400 million years for progenitor white dwarfs to form) = 13 billion years look-back time.
How does this comport with the 13.8 billion year clock for the age of the universe?
We see no evidence of Type Ia supernovae in galaxies with redshifts greater than 4. These galaxies and the very young univserse simply weren’t old enough at 800 million years for white dwarfs to have formed.
Penzias and Wilson and the Cosmic Microwave Background
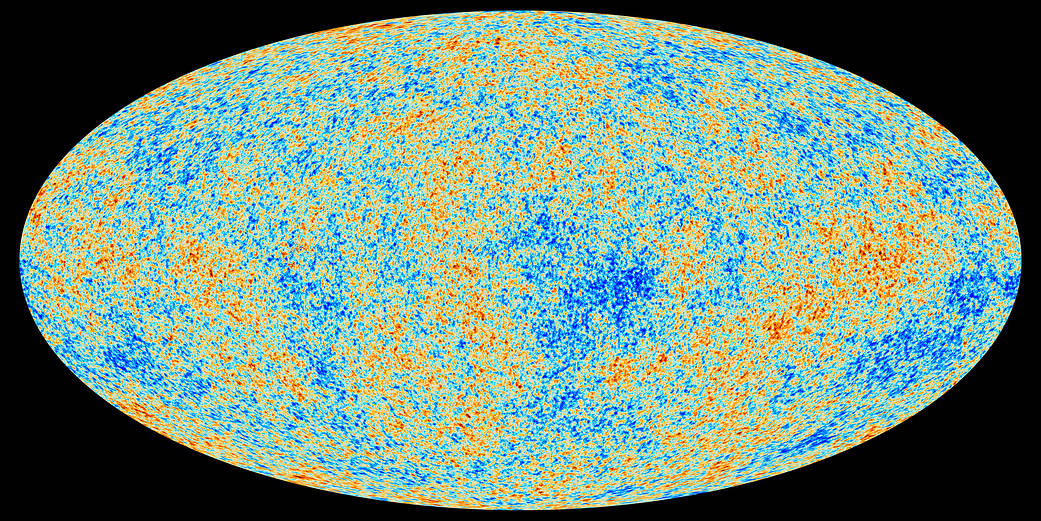
This intenstiy map of the Cosmic Microwave Backgroundd shows the oldest light in our universe, as detected with the greatest precision yet by the Planck mission. The ancient light, called the cosmic microwave background, was imprinted on the sky when the universe was 370,000 years old. It shows tiny temperature fluctuations that correspond to regions of slightly different densities, representing the seeds of all future structure: the stars and galaxies of today.
Image credit: ESA and the Planck Collaboration, Aug 7, 2017
In 1963 Arno Penzias and Robert Wilson, 2 Engineers at Bell Labs, tried in vain to elimate “Background Noise” from the radar systems they were working on. Their painstaking research ultimately led to the scientific discovery of the decade, a discovery that would earn them the 1978 Nobel Prize in Physics. Not only did they receive the Nobel Prize for their discovery, it was featured in the American Physical Society’s This Month in Physics History for June.
Over the intervening decades since the 1963 discovery, the initial age of 15 billion years has been refined to 13.77 billion years, a determination made through the success of 3 NASA missions (COBE, WMAP, PLANCK) and the painstaking work of thousands of researchers. This research now continues with the Atacama Cosmology Telescope (ACT).
1978 Nobel Prize in Physics
Quoting from the 1978 Nobel Prize press release, reference is made to “very high temperatures”, temperatures that allow nucleosynthesis to occur in the open environment. These thermonuclear fusion reactions produced what is known today as the “Priomordial Abundance” of hydrogen, helium, lithium and berylillium. The exapanding gas continued to cool and the process abated, forever etching in stone the total amount of matter the universe will ever have.
A theory developed by the American physicist Gamow and his associates suggested that this synthesis took place at the beginning of the existence of the universe. It is known from studies of the spectra of stars and galaxies that the universe is at present expanding uniformly. This means that at a certain point, 15 billion years ago, the universe was very compact; it is thus tempting to assume that the universe was created by a cosmic explosion, or ‘big bang’, although other explanations are possible. This ‘big bang’ theory implies the occurrence of very high temperatures, of about 10 billion degrees. Only at those temperatures can various nuclear reactions take place such that chemical elements could be built up from the elementary particles assumed to be present from the very beginning. It also implies the release of a large amount of radiation, whose spectrum extends from the X-ray region, through visible light, to radio waves. After this hypothetical explosion, the temperature would decrease rapidly (the whole ‘creation’ is assumed to have been completed in a few minutes).
Known as an almost perfect Blackbody Radiator, the Cosmic Microwave background (CMB) is the cooling afterglow of the nascent universe, 380,000 years after it began. At the point when this light we see today as the CMB first became visible, the expanding and cooling material became transparent, allowing its photons to begin their long journey accros space and time. We refer to this as the “Recombination Epoch“.
From our previous article: James Webb Space Telescope Deep Field Confirms Evolutionary Theories of Early Universe
The Recombination Epoch
The Recombination Epoch begins at about 18,000 years as electrons combine with helium nuclei (Alpha particles) to form He+. After 100,000 years, neutral helium forms and after 370,000 years, neutral hydrogen atoms (one proton, one electron) form (recombine).
As this epoch begins, the cooling plasma was still too hot to allow neutral atoms to form and thus, existed as an opaque, ionized gas. The ionized gas was hot -and opaque- and thus, no light could travel through it. This epoch concludes after 380,000 years with the formation of neutral hydrogen. At this point the universe becomes transparent for the first time.
The newly-formed atoms, mostly hydrogen and helium with traces of lithium, quickly reach their ground state energy by releasing photons. These photons are observed today as the cosmic microwave background (CMB).
The CMB
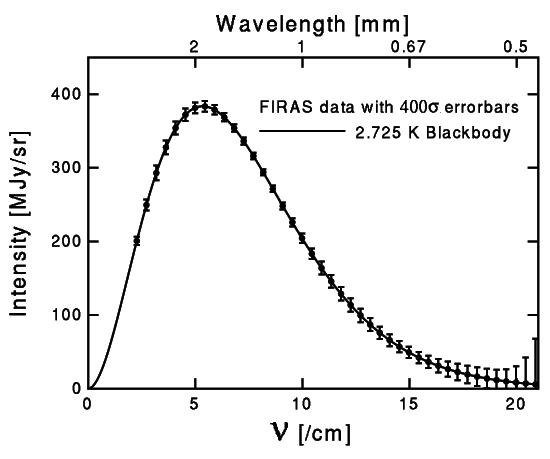
The power spectrum of the CMB as measured in 1990 by the FIRAS instrument aboard COBE. It is the most perfect blackbody spectrum in nature ever observed. The error bars are four hundred times larger than normal, just so one can see them!
Any object that has a temperature emits light. The hotter the object, the shorter the wavelength of light emitted. The sun, with a 5780 K “surface” temperature, for example, emits most of its energy in the yellow-green part of the visible spectrum. We call any object that behaves this way a Blackbody. A blackbody will absorb all incident light and reflect none. At some point, this object will arrive at an equilibrium point between the energy received and the energy emitted. The light thus emitted only depends on its “effective temperature“. Refering back to our example, the sun’s effective temperature is 5780 degrees Kelvin or 5780 K.
When we look at the Cosmic Microwave Background, the oldest, purest light in the universe, we’re seeing the universe as it was the instant in time photons were able to break free of the 2,700 Kelvin, opaque plasma that kept them bound. At that moment, having cooled sufficiently, the universe became transparent. This point is known as the “time of last scattering” when the universe became transparent to electromagnetic radiation. We’re seeing that sky today, not at 2,700 k but at 2.7 K. Why?
The Microwave Sky
Arno Penzias and Robert Wilson detected this ancient light not as a blinding heat flash (a 2,700 K Blackbody emits most of its energy in the near infrared) but as mm-wave white noise in their radio receiver. To them, using the radio technology available in 1963, the microwave sky was isotropic and uniform no matter where they pointed the antenna. Over the intervening decades since, with the success of 3 NASA missions (COBE, WMAP, PLANCK) and work now being conducted with the Atacama Cosmology Telescope (ACT), fine anisotropies (irregularities) in the CMB have been detected. These irregularities, much less than 0.001 degrees K, can be seen as variations in color in the (microwave sky map) image above. Of all the indicators of the Universe’s age, the CMB is the most definative.
The Cosmological Red Shift and the Age of the Universe
The 2.7 K background we see today is really the 2,700 K background of the nascent universe 13.769 billion years ago.
Due to Cosmological Red Shift, the stretching out of space with distance and, dating back to just 380,000 years after the Big Bang, the power spectrum of the CMB is redshifted 1000 times into the millimeter-wave region of the Electromagnetic spectrum! We see the 2,700 K light immediatley following Recombination, redshifted 1000 times!
The magnitude of this shift is a direct consequence of its age according to the Hubble Constant, and is as empirical as it gets.
The age of the universe is etched in stone by the CMB at 13.77 Billion years.
Unlocking the CMB has been Cosmology’s Holy Grail and has permitted us to thus far tell the story of the nascent universe with unprecidented detail. In addition to the bedrock empirical determination of the universe’s age described above, a recent study conducted with the Atacama Cosmology Telescope (ACT), the separation of the micro-changes in temperature is also used to confirm the age of the universe. That paper, part of a five-part study, can be found here.
Globular Clusters and Methuselah’s Star, the Oldest Known Star
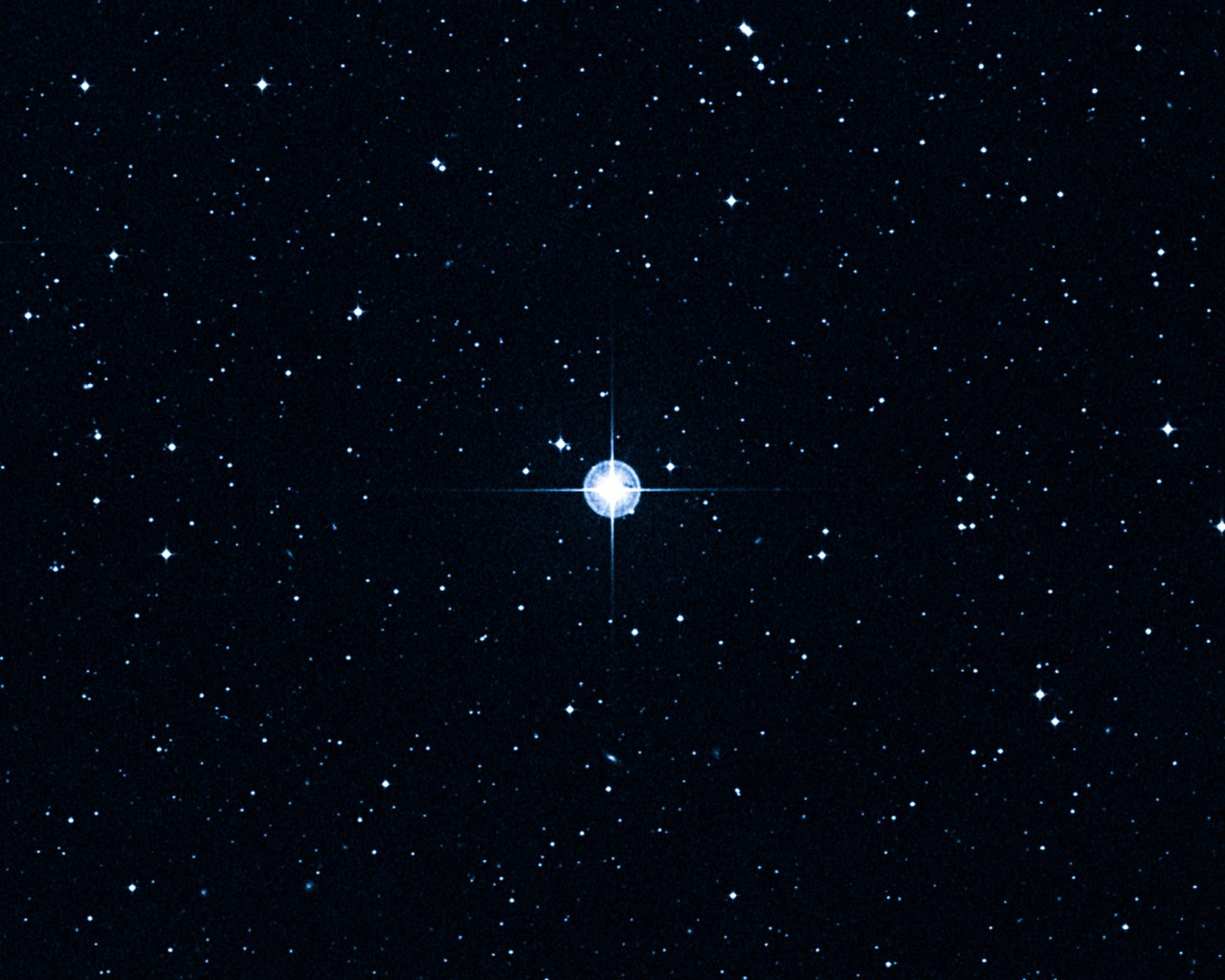
Digitized Sky Survey image of Methuselah’s Star, the oldest star with a well-determined age in our galaxy. The aging star, cataloged as HD 140283, lies 202 light-years away in the constellation Libra. The NASA/ESA Hubble Space Telescope was used to narrow the measurement uncertainty on the star’s distance, and this helped refine the calculation of a more precise age. The star’s orbit carries it through the plane of our galaxy from the galactic halo that has a population of ancient stars. Image credit: The Anglo-Australian Observatory (AAO) UK Schmidt telescope.
Basic logic would dictate that you wouldn’t expect to find stars older than the universe in which they reside.
That we don’t find any stars older than 14 Gyr suggests that this is the upper age limit for the universe. Granted, there are 200+ billion stars in our galaxy alone and it would be impossible to age them all but, statistically, we’re not going to find any stars older than this for one simple reason:
Regardless of what galaxy they reside in, the first stars’ metalicity ratios, the ratio of metals (anything heavier than hydrogen or helim) to hydrogen or helium, would have to be consistent with the primordial abundance. This one property of a star, its metalicity, sets its age in stone and cannot be contradicted by any other determination.
Methuselah’s Star
To date, the oldest known star is Methuselah’s star or HD 140283 (Henry Draper catalog) in Libra.
In a universe older than say ~ 15 or 16 Gyr, the primordial abundance would be much different and thus, inconsistent with what is observed in Methuselah’s star. The abunances observed in this star are inconsistant with an older universe and set the age of the universe at 13.77 Gyr.
Using the fine guidance sensor of the Hubblee Space Telescope, the parallax distance for HD 140283 (Methuselah’s star) was determined to be 202.4 ± 0.9 ly as published in a comprehensive 2013 study. Quoting from the study’s abstract, we note “the age of HD 140283 does not conflict with the age of the Universe, 13.77 ± 0.06 Gyr, based on the microwave background and Hubble constant, but it must have formed soon after the big bang.”
The quoted error includes only the uncertainty in the parallax, and is for adopted surface oxygen and iron abundances of [O/H] = -1.67 and [Fe/H] = -2.40. Uncertainties in the stellar parameters and chemical composition, especially the oxygen content, now contribute more to the error budget for the age of HD 140283 than does its distance, increasing the total uncertainty to about ±0.8 Gyr. Within the errors, the age of HD 140283 does not conflict with the age of the Universe, 13.77 ± 0.06 Gyr, based on the microwave background and Hubble constant, but it must have formed soon after the big bang.
Globular Clusters
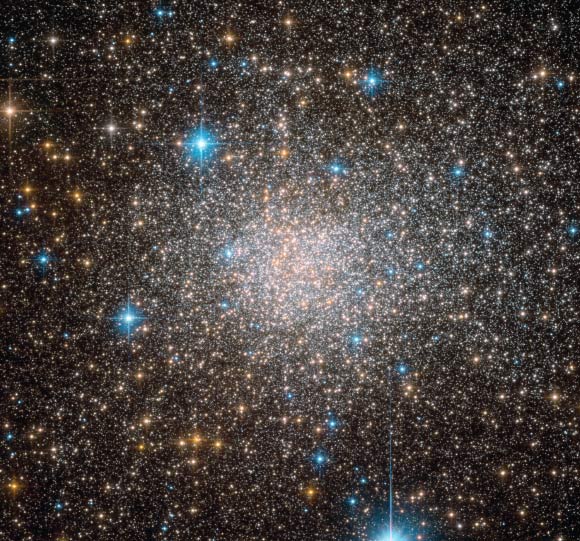
The globular cluster and galactic relic Terzan 5 in Sagittarius. The bright, red-orange stars are the old, evolved Pop II stars that date back to the early universe. Image credit: F. Ferraro / NASA / ESA / ESO.
To date, the oldest stars found reside in Globular Clusters, huge agglomerations of at least 100,000 stars orbiting the galactic center in what is known as the Galactic Halo. In a previous article about one such relic, Terzan 5 in Sagittarius, we discuss it in detail.
Quoting from that article
I’m fascinated with relics as they have a story to tell, a deep story of something very old, of a long-gone epoch, a bridge, if you will, back to a time when the universe was much younger -and much different. Globular clusters are populated with very old stars, each a relic unto itself with a story to tell of a time when the universe was much younger.
and
It is a globular star cluster set against the galactic center in Sagittarius, a cluster where we observe the very same type of ancient stars, stars such as Arcturus, stars that have evolved, exhausting their compliment of hydrogen and have begun helium fusion.
and finally:
This object resembles a globular cluster, but is like no other such object, and is in fact one of the Milky Way’s galactic bulge’s primordial building blocks, most likely a relic from our galaxy’s early history. Terzan 5 contains two distinct populations of stars, younger Pop I and II stars with ages at 4.5 billion years (Gyr) and ancient stars with ages greater than 12 billion years (Gyr). The former population of stars are at similar ages as the sun whilst the older stars, the late Pop. II stars, date back to the very first stars formed, highlighting two distinct periods of star formation separated by 7 billion years.
That globular clusters inhabit the galactic halo, a region surrounding the galactic center and replete with the oldest known stars in the galaxy, suggests an age for them consistent with the first stars to have formed.
Conclusion
We have presented 3 key points that converge on the age of the universe with a high concordance:
- Type Ia Supernovae
- The Cosmic Microwave Background (CMB)
- The Oldest Stars and Globular Clusters
We thus conclude its age, determined by hundreds of studies, 3 NASA missions, 2 Nobel Prizes, refined by thousands of scholars over 9.4 decades, to be 13.77 Gyr.
Astronomy For Change: https://astronomyforchange.org
Did you enjoy this article or like what we do? Why not leave a tip or buy us a Coffee?
Follow Us On Twitter: https://twitter.com/astronomychange
Why not support us on Patreon: https://www.patreon.com/astronomyforchange
Imagination is more important than knowledge
An index of all articles can be found here.
If you enjoyed this article, please consider supporting us with a modest donation
or through a subscription on our Patreon Page
Membership at Astronomy for Change is Free!
well now, this article aged like a feminist hitting 30