Like early explorers mapping the continents of our globe, astronomers are busy charting the spiral structure of our galaxy, the Milky Way. Using infrared images from NASA’s Spitzer Space Telescope, scientists have discovered that the Milky Way’s elegant spiral structure is dominated by just two arms sweeping off the ends of a central bar of stars. These observations confirm the widely-held view that the Milky Way is of the variety of galaxy known as a “Barred Spiral”. Previously, our galaxy was thought to possess four major arms. In this annotated artist’s concept illustration, the new view of the Milky Way is presented. The galaxy’s two major arms (Scutum-Centaurus and Perseus) can be seen attached to the ends of a thick central bar, while the two now-demoted minor arms (Norma and Sagittarius) are less distinct and located between the major arms. The major arms consist of the highest densities of both young and old stars; the minor arms are primarily filled with gas and pockets of star-forming activity. The artist’s concept also includes a new spiral arm, called the “Far-3 kiloparsec arm,” discovered via a radio-telescope survey of gas in the Milky Way. This arm is shorter than the two major arms and lies along the bar of the galaxy. Our sun lies near a small, partial arm called the Orion Arm, or Orion Spur, located between the Sagittarius and Perseus arms.
Ever since Edwin Hubble determined that the spiral nebulae were separate galaxies in 1924, specifically the great spiral galaxy in Andromeda, that these objects were separate agglomerations of stars numbering in the tens to hundreds of billions or trillions for some galaxies, that they were separate and apart from the Milky Way galaxy, precise determination of their mass was then and remains today a key piece of the large-scale cosmological picture, theories on galaxy formation and the evolution of the Universe. To that end, astronomers have employed various techniques for measuring the true mass of the Milky Way.
Adding an additional level of complexity to the mix is the contribution of dark matter. More than anything else, the term “dark matter” describes the observed effects of an unseen mass rather than the true nature of that mass with its intrinsic properties remaining an open question. Dark matter has never been directly observed or measured; however, as mentioned previously, the term suggests it is not observable and hence, does not emit or interact with electromagnetic radiation, such as light, and is thus invisible to the entire electromagnetic spectrum. Why is this important?
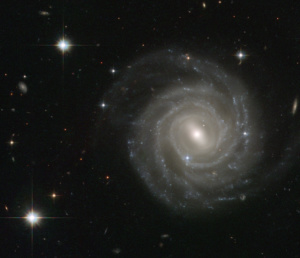
The galaxy in this stunning image captured by the NASA/ESA Hubble Space Telescope, UGC 12158, is an excellent example of a barred-spiral galaxy and is thought to be a good likeness of our home galaxy, the Milky Way. Note the prominent blue “star” below and to the left of the galaxy’s center. This “star” is a Type Ia supernova, SN2004ef.
Using galaxy rotation curves in the 1960s and 1970s, Vera Rubin and Kent Ford demonstrated that the observed rotational dynamics of our galaxy and other spiral galaxies is inconsistent with a 1/r dependence beyond a certain distance from the center. The 1/r dependence on rotation is what we observe in our coffee after adding creamer and stirring; this resulting spiral pattern is a natural consequence of a 1/r dependence. For the Milky Way, the path of the sun’s orbit around the center, known as the Local Standard of Rest, is coincidentally the point beyond which the Milky Way rotates as though there is a constant mass [beyond the Local Standard of Rest]. This behavior and similar behavior observed in other galaxies is ascribed to dark matter as the observed or “baryonic” mass cannot account for the rotational speed.
Why choose the Milky Way? Well, for starters, the Milky Way is our home galaxy, by all accounts a large, barred-spiral galaxy whose mass is of the order of 100 – 400 billion stars. Being part of a system (eg. earth as part of the solar system or leveraging our proximity to the sun to model other stars) provides a unique vantage point. We observe other spiral galaxies and test our theories accordingly but, because of the tremendous distances involved, we are unable to observe them with the necessary resolution; even the nearest galaxies (eg. the Great Galaxy in Andromeda), only the most luminous stars are resolvable as point sources – this limit is the catalyst that drives each new generation of telescope (HST). Being part of the system under study provides a crucial advantage, allowing us to resolve the system’s behavior with the necessary detail.
When we look up at the stars at night, even over the course of a human lifetime, observing any appreciable change in the relative position of all but the nearest stars would be impossible without a telescope. This 3-D motion is known as “proper motion” or motion relative to our location. That motion is controlled, principally, by the mass of the galaxy and thus, a more precise determination of a given star’s proper motion and velocity will allow us to more tightly constrain the mass of the galaxy. In a classic sense, the galaxy can be modeled as an agglomeration of billions of stars, each star in orbit around a common center, an object whose mass is the gravitationally bound aggregate of all the member stars. The precise determination of that aggregate mass is the object of this study.
Circular motion is well understood and is taught to all physics and engineering students from high school freshman to Ph.D students. The principles that govern the motion of an internal combustion engine’s flywheel or the rotation of an amusement park ride are the same that govern the rotational motion of a spiral galaxy. The only difference is the size, scale, velocities and masses involved. Simply put, the observed rotational velocities of the Milky Way and other spiral galaxies are inconsistent with their observed masses.
As stated above, only the most luminous stars are resolvable as point sources in the nearest galaxies and thus the number of stars whose proper motions are observable is severely limited. Not so for the Milky Way; the proper motions of many thousands of stars is well catalogued and thus, the motion of those stars could be used to determine the mass of the Milky Way.
Once a more precise value for the Milky Way’s mass is determined, we can use our home galaxy as a standard and thus refine our models of galactic formation and evolution. In classical orbital and rotational dynamics, the rotational speed, the distance from the center of the gravitating mass (the galaxy’s mass, in this case) and the gravitating mass are tightly linked, one depends upon the other; if you change one, the dynamics of the whole system change. You may ask, well, why don’t we use the orbital period of the sun around the galaxy and, since we know the distance to the Milky Way’s center (~25 K Ly), we can calculate the mass of the galaxy? The problem with using this classic technique is that the proper motions of the stars observed are inconsistent with the observed mass of the galaxy and thus a different approach needs to be used, one that accounts for the unseen mass or Dark Matter.
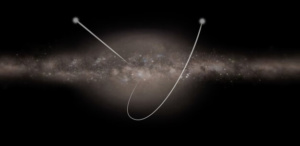
The trajectories of two HVSs are depicted in this cross-sectional illustration of the Milky Way’s galactic disk.
All matter, seen and unseen, will affect the trajectory of an object moving in a radial direction with respect to the galactic center and thus, will not be subject to orbital velocity anomalies linked to unseen Dark Matter in the outer galactic disk. Enter Dr. Giacomo Fragione, an astrophysicist at the University of Rome, and Professor Abraham Loeb – the Frank B. Baird, Jr. Professor of Science, the Chair of the Astronomy Department, and the Director of the Institute for Theory and Computation at Harvard University. In a recently published study in Astronomy and Astrophysics, Drs. Fragione and Baird used Hypervelocity stars whose radial trajectories and velocities will result in their permanent departure from the galaxy. Using classical gravitational theory, the masses of these stars, their velocities and distances from the center, it is easy to constrain the upper limit of the Milky Way’s mass. Said differently, their velocities exceed the escape velocity of the Milky Way. Although the motions of these Hypervelocity Stars (HVSs) are technically their “proper motion”, they are defined as stars able to escape the Milky Way’s gravity well. Observed to be traveling in excess of 700 km/sec (0.2% C, the speed of light!), they derived their high speeds and tremendous kinetic energy from interactions with the supermassive black hole at the galactic center.
In conclusion, the study found that the Milky Way’s upper mass is constrained at 1.2 – 1.9 trillion (1 x ten to the twelfth power) solar masses! This doesn’t mean that there are 1.2 – 1.9 trillion stars in the Milky Way; this number is an upper limit constraining the total mass of the galaxy. The total number of stars is determined by using the average stellar mass for all the stars in the galaxy after all other contributions to the total mass of the galaxy have been accounted for. Remember, this is the total mass of the galaxy, mass that includes the aggregate stellar population, the Interstellar Medium, the gas and dust between the stars and Dark Matter.
If we adopt the widely-held percentage of Dark Matter to “regular” or baryonic mass of 90%, we find that Dark Matter accounts for 1.1 – 1.7 trillion solar masses with only 120 – 190 billion solar masses remaining for stars, gas and dust. If we adopt a value of 20% of the remaining mass for the Interstellar Medium (gas and dust) or 24 – 38 billion solar masses, the remaining 80% or 96 – 152 billion solar masses account for the aggregate stellar mass.
Most stars in the galaxy, about 70%, are one solar mass or less. Using an average stellar mass of 0.5 solar masses, the new result puts the total number of stars in the galaxy at 192 – 300 billion. This result is consistent with estimations to date. What this result does is to put an upper limit on the Milky Way’s mass and answers a long-standing question that dates from 1924 and Edwin Hubble’s discovery that the universe is an endless, expanding ocean of space and time!
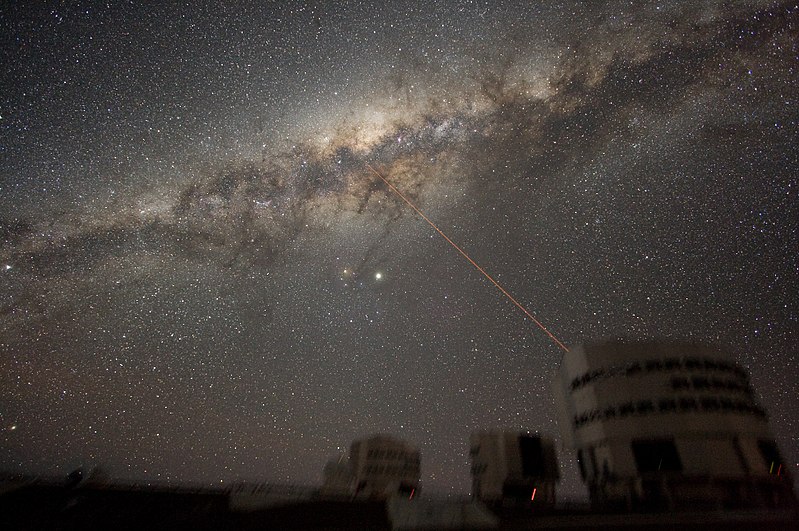
Image of the night sky and the Milky Way’s Galactic Center above Paranal on 21 July 2007, taken by ESO astronomer Yuri Beletsky. A wide band of stars and dust clouds, spanning more than 100 degrees on the sky, can be seen. At the center of the image, two bright objects are visible. The brightest is the planet Jupiter, while the other is the star Antares. Three of the four 8.2-m telescopes forming ESO’s VLT (Very Large Telescope) are seen, with the guide laser from the telescope’s Adaptive Optics system beaming out from Yepun, Unit Telescope number 4. The laser is pointing directly at the Galactic Center. Also visible are three of the 1.8-m Auxiliary Telescopes used for interferometry. The exposure time is 5 minutes and since the camera is tracking the stars as the earth rotates, the telescopes are slightly blurred.
Woah! I’m really enjoying the template/theme of this website.
It’s simple, yet effective. A lot of times it’s hard
to get that “perfect balance” between user friendliness and
visual appearance. I must say you have done a awesome job with this.
Also, the blog loads very quick for me onn Firefox.Outstanding Blog!